Characterizing Cosmic Neutrino Sources
A Measurement of the Energy Spectrum and Flavor Composition of the Cosmic Neutrino Flux Observed with the IceCube Neutrino Observatory
Added: July 26, 2022.
Rather than in high-energy gamma astronomy, I carried out my PhD project in the - closely related - field of high-energy neutrino astronomy. From 2012 to 2015, I joined the IceCube Collaboration, which runs one of the most fascinating detectors that was ever built: the IceCube Neutrino Observatory, situated at the geographical South Pole in Antarctica.
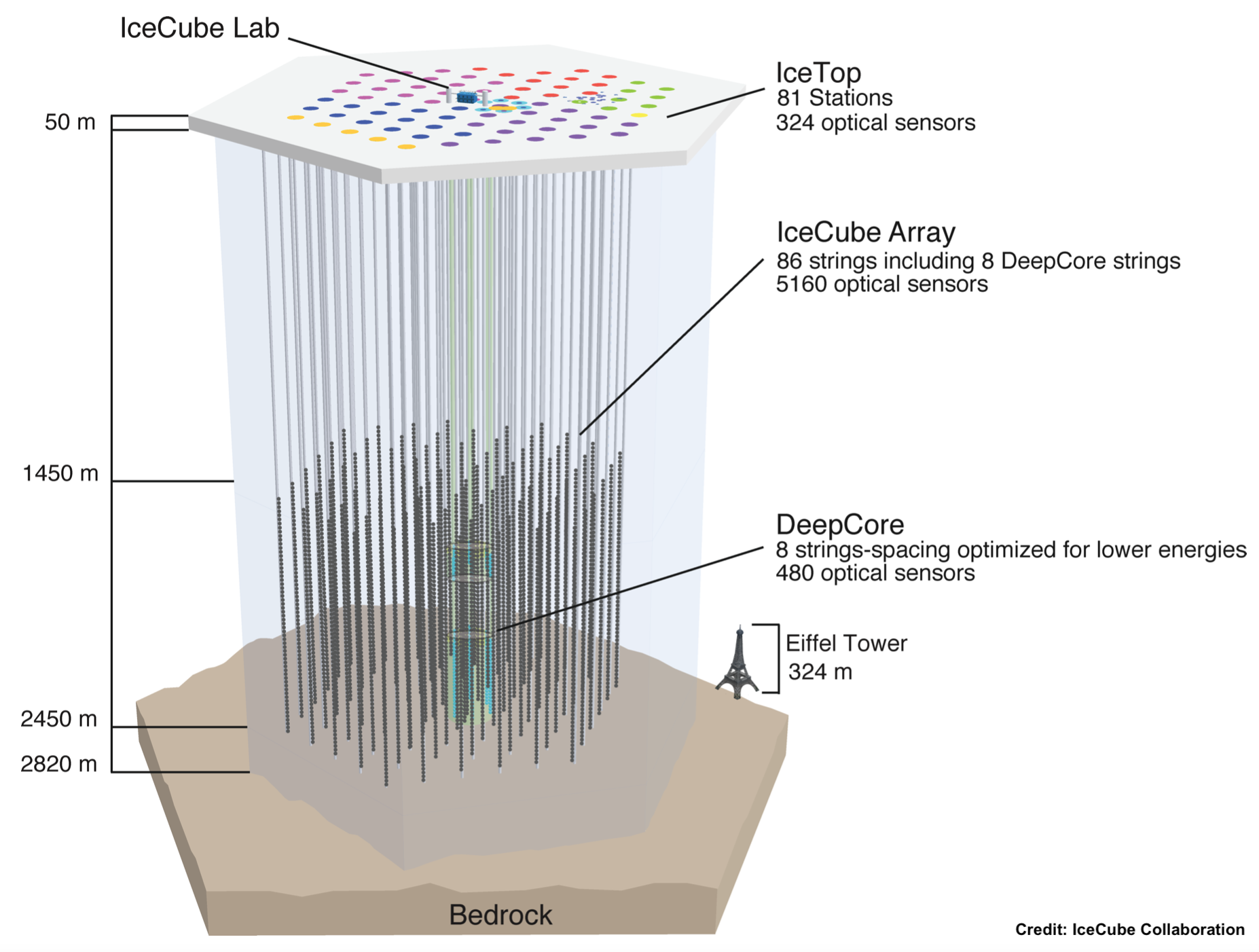
The IceCube detector consists of more than 5,000 optical sensors, frozen deep into the Antarctic glacier, and instrumenting a total volume of about a cubic kilometre. When neutrinos undergo scattering within or close to the detector, secondary particles are created, which radiate so-called Cherenkov light when they travel through the ice. IceCube’s optical sensors detect this light, and from the combined signals, it is possible to infer the properties of the original neutrino.
In 2013, the IceCube Collaboration announced the discovery of a flux of high-energy cosmic neutrinos. Such a flux had long been sought for, but, due to the extremely feeble interaction of neutrinos with matter, it required a detector the size of IceCube to finally detect it. The cosmic neutrino flux is diffuse, that is, it appears to arrive isotropically from all directions, and its sources are not fully uncovered to this day.
Nevertheless, I set out to characterize the sources of these neutrinos. This is possible by studying two intrinsic properties of the cosmic neutrino flux: its energy spectrum, and its flavour composition.
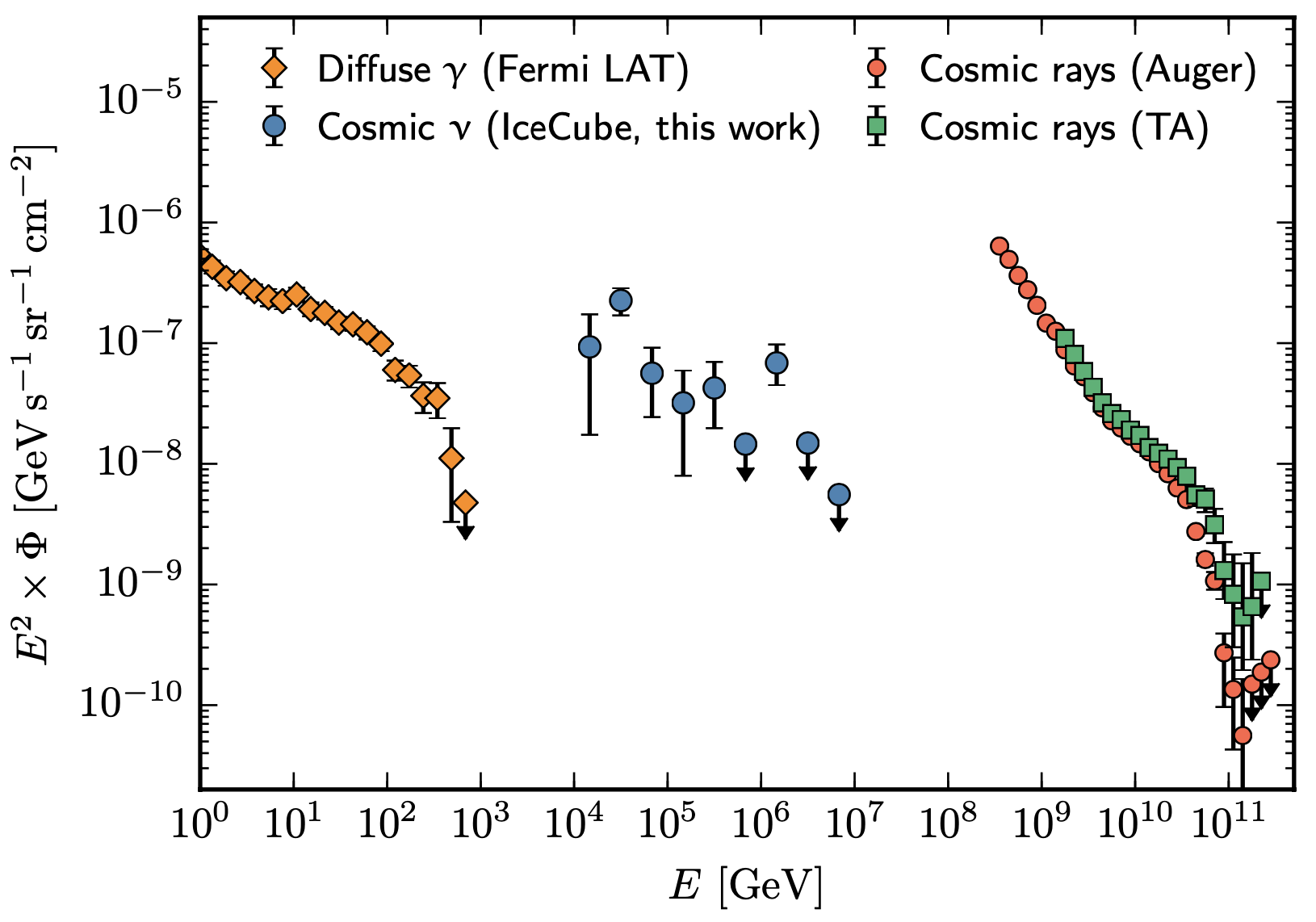
The measured energy spectrum of the cosmic neutrino flux is shown in the plot on the left, and compared to the diffuse fluxes of gamma rays and charged cosmic rays. The plot shows that the total energy carried by cosmic neutrinos equals that of diffuse gamma rays at around 100 GeV and that of cosmic rays at about 109 GeV. This demonstrates that neutrinos play an important role in the high-energy Universe, and suggests that the origin of gamma rays, neutrinos, and cosmic rays are linked (as has long been suggested from theoretical arguments).
On the other hand, the flavour composition of the cosmic neutrino flux designates its separation into the three neutrino flavours: electron neutrinos (νe), muon neutrinos (νμ), and tau neutrinos (ντ). It is expected that at their sources, cosmic neutrinos are produced as electron or muon neutrinos, but not as tau neutrinos. Due to neutrino flavour oscillations, however, the flavour composition changes during the propagation of the neutrinos from their sources to Earth. Nevertheless, the composition measured at Earth can provide constraints on the composition at the sources.
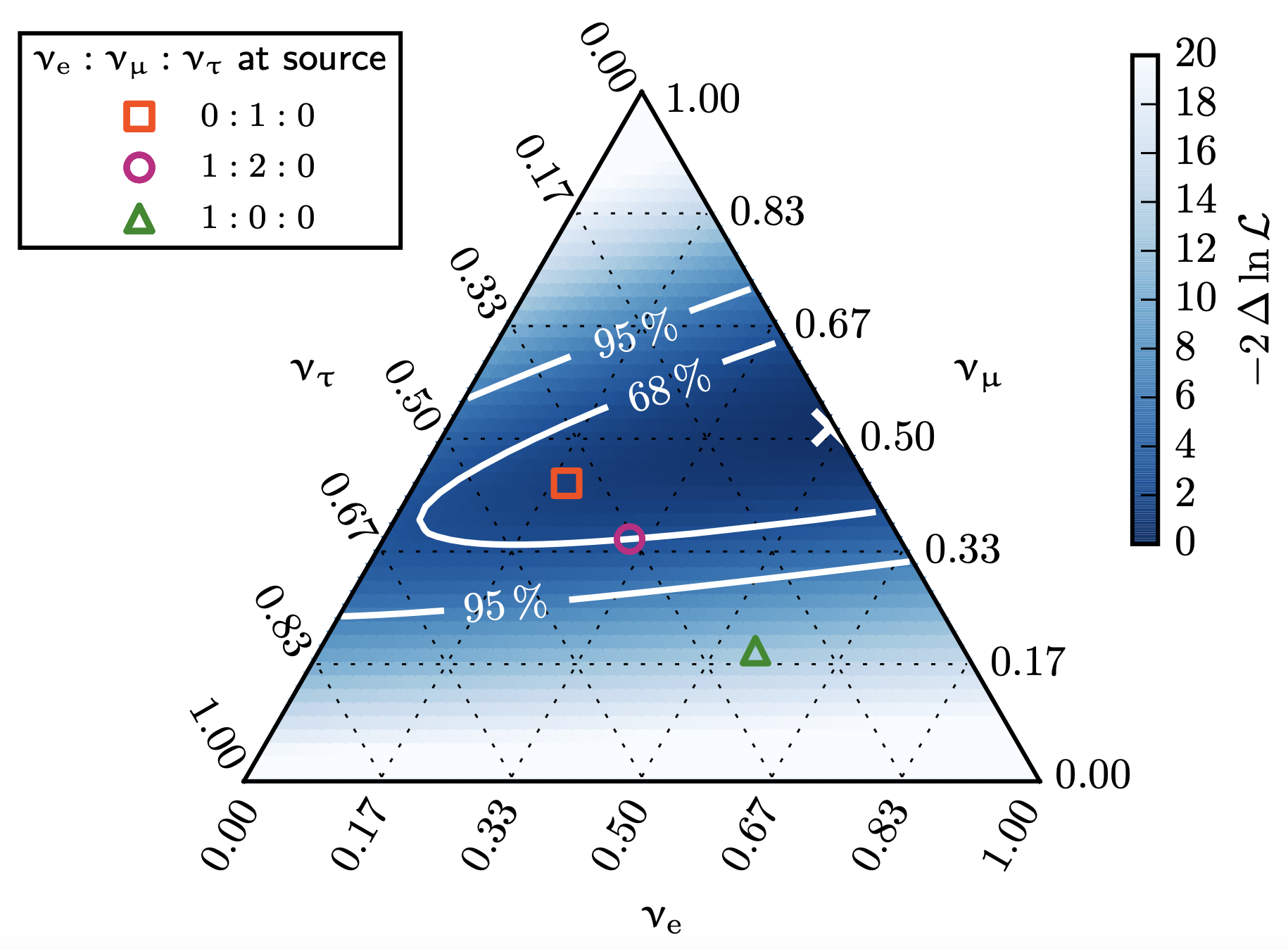
The measured flavour composition can be displayed in a triangular plot, as shown on the right. Here, each of the three axes denotes the fraction of each of the three flavours in the total cosmic neutrino flux. While the analysis yields a best-fit composition of 50% electron and muon neutrinos each, many other compositions are still allowed (as shown by the white confidence lines). However, it was possible to exclude a scenario in which only electron neutrinos are created at the sources (as would be expected from the decay of neutrons).
The results of my PhD work have been published in an article by the IceCube Collaboration: ApJ 809, 98 (2015).